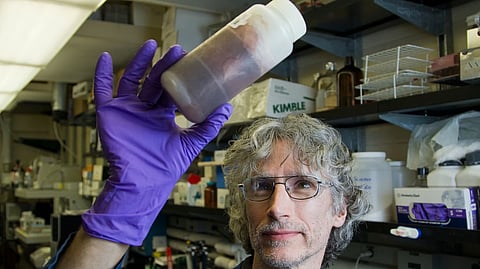
But is all that variety necessary? Could biology work just as well with fewer building blocks and simpler polymers?
Ting Xu, a University of California, Berkeley, polymer scientist, thinks so. She has developed a way to mimic specific functions of natural proteins using only two, four or six different building blocks — ones currently used in plastics — and found that these alternative polymers work as well as the real protein and are a lot easier to synthesize than trying to replicate nature's design.
As a proof of concept, she used her design method, which is based on machine learning or artificial intelligence, to synthesize polymers that mimic blood plasma. The artificial biological fluid kept natural protein biomarkers intact without refrigeration and even made the natural proteins more resistant to high temperatures — an improvement over real blood plasma.
The protein substitutes, or random heteropolymers (RHP), could be a game-changer for biomedical applications, since a lot of effort today is put into tweaking natural proteins to do things they were not originally designed to do, or trying to recreate the 3D structure of natural proteins. Drug delivery of small molecules that mimic natural human proteins is one hot research field.
Instead, AI could pick the right number, type and arrangement of plastic building blocks — similar to those used in dental fillings, for example — to mimic the desired function of a protein, and simple polymer chemistry could be used to make it.
In the case of blood plasma, for example, the artificial polymers were designed to dissolve and stabilize natural protein biomarkers in the blood. Xu and her team also created a mix of synthetic polymers to replace the guts of a cell, the so-called cytosol. In a test tube filled with artificial biological fluid, the cell's nanomachines, the ribosomes, continued to pump out natural proteins as if they didn't care whether the fluid was natural or artificial.
"Basically, all the data shows that we can use this design framework, this philosophy, to generate polymers to a point that the biological system would not be able to recognize if it is a polymer or if it is a protein," said Xu, UC Berkeley professor of chemistry and of materials science and engineering. "We basically fool the biology. The whole idea is that if you really design it and inject your plastics as a part of an ecosystem, they should behave like a protein. If the other proteins are like, 'Okay, you are part of us,' then that's OK."
The design framework also opens the door to designing hybrid biological systems, where plastic polymers interact smoothly with natural proteins to improve a system, such as photosynthesis. And the polymers could be made to naturally degrade, making the system recyclable and sustainable.
"You start to think about a completely new future of plastic, instead of all this commodity stuff," said Xu, who is also a faculty scientist at Lawrence Berkeley National Laboratory.
She and her colleagues published their results in the March 8 issue of the journal Nature.
A happy mix of biological and abiological polymers
Xu sees living tissue as a complex mix of proteins that evolved to work together flexibly, with less attention paid to the actual amino acid sequence of each protein than to the functional subunits of the protein, the places where these proteins interact. Just as in a lock-and-key mechanism, where it doesn't make much difference whether the key is aluminum or steel, so the actual composition of the functional subunits is less important than what they do.
And since these natural protein mixtures evolved randomly over millions of years, it should be possible to create similar mixtures randomly, with a different alphabet of building blocks, if you use the right principles to design and select them, relieving scientists of the need to recreate the exact protein mixtures in living tissue.
That randomness can be leveraged to design synthetic polymers that mix well with natural proteins, creating biocompatible plastics more easily than today's targeted techniques, Xu says.
Working with applied statistician Haiyan Huang, a UC Berkeley professor, the researchers developed deep learning methods to match natural protein properties with plastic polymer properties in order to design an artificial polymer that functions similarly, but not identically, to the natural protein. For example, in trying to design a fluid that stabilizes specific natural proteins, the most important properties of the fluid are the electric charges of the polymer subunits and whether or not these subunits like to interact with water — that is, whether they are hydrophilic or hydrophobic. The synthetic polymers were designed to match those properties, but not other characteristics of the natural proteins in the fluid.
Huang and graduate student Shuni Li trained the deep learning technique — a hybrid of classical artificial intelligence (AI) that Huang refers to as a modified variational autoencoder (VAE) — on a database of about 60,000 natural proteins. These proteins were broken down into 50-amino acid segments, and the segment properties were compared to those of artificial polymers composed of only four building blocks.
With feedback from experiments by graduate student Zhiyuan Ruan in Xu's lab, the team was able to chemically synthesize a random group of polymers, RHPs, that mimicked the natural proteins in terms of charge and hydrophobicity.
"We look at the sequence space that nature has already designed, we analyze it, we make the polymer match to what nature already evolved, and they work," Xu said. "How well you follow the protein sequence determines the performance of the polymer you get. Extracting information from an established system, such as naturally occurring proteins, is the easiest shortcut to enable us to tease out the right criteria for creating biologically compatible polymers."
Colleagues in the lab of Carlos Bustamante, UC Berkeley professor of molecular and cell biology, of chemistry and of physics, performed single molecule optical tweezers studies and clearly showed that the RHPs can mimic how proteins behave.
Xu, Huang and their colleagues are now trying to mimic other protein characteristics to reproduce in plastic the many other functions of natural amino acid polymers.
"Right now, our goal is simply stabilizing proteins and mimicking the most basic protein functions," Huang said. "But with a more refined design of the RHP system, I think it's natural for us to explore enhancing other functions. We are trying to study what sequence compositions can be informative regarding the possible protein functions or behavior that the RHP can carry."
The design platform opens the door to hybrid systems of natural and synthetic polymers, but also suggests ways to more easily make biocompatible materials, from artificial tears or cartilage to coatings that can be used to deliver drugs.
"If you want to develop biomaterials to interact with your body, to do tissue engineering or drug delivery, or you want to do a stent coating, you have to be compatible with biological systems," Xu said. "What this paper is telling you is: Here are the design rules. This is how you should interface with biological fluids."
Her ultimate goal is to totally rethink how biomaterials are currently designed, because current methods — focused primarily on mimicking the amino acid structures of natural proteins — are not working.
"The Food and Drug Administration hasn't approved any new material for polymer biomaterials for decades, and I think the reason is that a lot of synthetic polymers are not really working — we are pursuing the wrong direction," she said. "We are not letting the biology tell us how the material should be designed. We are looking at individual pathways, individual factors, and not looking at it holistically. The biology is really complicated, but it's very random. You really have to speak the same language when dealing with materials. That's what I want to share with the materials community." (PB/Newswise)